Fabricación y caracterización de pozos cuánticos para el estudio de la interacción luz-materia
DOI:
https://doi.org/10.29059/cienciauat.v17i2.1737Palabras clave:
pozo cuántico, espectroscopía, excitonesResumen
Los pozos cuánticos representan la base de una gran variedad de dispositivos electrónicos, entre ellos los ledes, láseres, fotodetectores y moduladores. Las propiedades optoelectrónicas de estos sistemas dependen de su composición química y del espesor de las capas crecidas. Por dicha razón, es necesario tener un control preciso durante su crecimiento. El presente trabajo tuvo como objetivo inducir el crecimiento epitaxial de pozos cuánticos intrínsecos (sin ningún dopaje) asimétricos acoplados y desacoplados de AlGaAs/GaAs/AlGaAs, así como, establecer su caracterización mediante técnicas de espectroscopía óptica, como lo son la reflectancia diferencial, también conocida como espectroscopía de reflectancia anisotrópica (RAS) y la fotoluminiscencia (PL). Se realizó un estudio experimental de las interacciones entre niveles de energía en los pozos cuánticos asimétricos acoplados. Este tipo de estructuras son especialmente interesantes por permitir la formación y observación, no solo de excitones directos, dentro del mismo pozo, sino también, de excitones y triones indirectos, que se forman entre electrones de un pozo y huecos de otro pozo vecino (Transiciones intra-QW). Se hicieron crecer tres pozos intrínsecos, basados en arseniuro de galio (GaAs): un pozo individual (desacoplado) y un par de pozos asimétricos acoplados, a través de epitaxia por haces moleculares (MBE). Se observó el efecto del rompimiento de simetría (de D2d a C2v) en las propiedades espintrónicas de la estructura, a través de PL y RAS, a una temperatura de ~ 30 K. Se lograron establecer las técnicas y métodos necesarios para el crecimiento de pozos cuánticos intrínsecos, que constituyen la base para la creación de dispositivos y estructuras más complejas. El uso de técnicas espectroscópicas permitió demostrar la presencia de anisotropías ópticas, que repercuten en el comportamiento del espín de los excitones en pozos cuánticos.
Citas
Biermann, K., Helgers, P., Crespo-Poveda, A., Kuznetsov, A., Tahraoui, A., Röben, B., …, and Grahn, H. (2021). In situ control of molecular beam epitaxial growth by spectral reflectivity analysis. Journal of Crystal Growth. 557(124): 125993. DOI: https://doi.org/10.1016/j.jcrysgro.2020.125993
Braun, W. (1999). Applied RHEED reflection high energy electron diffraction during crystal growth. Springer Tracts in Modern Physics 154. New York: Springer-Verlag W. Braun. 216 Pp.
Braun, W., Trampert, A., Däweritz, L., and Ploog K. H. (1997). Nonuniform segregation of Ga at AlAs/GaAsheterointerfaces. Physical Review B. 55(3): 1689-1695. DOI: https://doi.org/10.1103/PhysRevB.55.1689
Bravo-Velázquez, C. A., Lastras-Martínez, L. F., Ruiz-Cigarrillo, O., Flores-Rangel, G., Tapia-Rodríguez, L. E., Biermann, K., and Santos, P. V. (2022). Photoluminescence of double quantum wells: Asymmetry and excitation laser wavelength effects. Physical Status Solidi B. 259(4): 2100612. DOI: https://doi.org/10.1002/pssb.202100612
Chen, Y., Cingolani, R., Andreani, L. C., and Bassani, F. (1988). Photoluminiscence in quantum well and bulk GaAs: a direct comparative study. Il Nuovo Cimento D. 10(7): 847-859. DOI: https://doi.org/10.1007/BF02450144
Downs, C. and Vandervelde, T. (2013). Progress in infrared photodetectors since 2000. Sensors (Basel, Switzerland). 13(4): 5054-5098. DOI: https://doi.org/10.3390/s130405054
Esser, A., Runge, E., Zimmermann, R., and Langbein, W. (2000). Photoluminiscence and radiative lifetime of trions in GaAs quantum wells. Physical Review B. 62(12): 8232-8239. DOI: https://doi.org/10.1103/PhysRevB.62.8232
Etienne, B. (1993). RHEED-based measurements of atomic segregation at GaAs/AlAs interfaces. Journal of Crystal Growth. 127(1-4): 1056-1058. DOI: https://doi.org/10.1016/0022-0248(93)90790-4
Guerra, N., Guevara, M., Palacios, C., and Crupi, F. (2018). Operation and physics of photovoltaic solar cells: An overview. Revista de I+D Tecnológico. 14(2): 84-95. DOI: https://doi.org/10.33412/idt.v14.2.2077
Hamaguchi, C. (2017). Basic semiconductor physics. Switzerland: Springer Nature. 426 Pp. DOI: https://doi.org/10.1007/978-3-319-66860-4
Harrison, P. and Valavanis, A. (2016). Numerical solutions. In: Quantum Wells, Wires and Dots: Theoretical and Computational Physics of Semiconductor Nanostructures. United Kingdom: John Wiley and Sons. 624 Pp. DOI: https://doi.org/10.1002/9781118923337
Harvey, T. E., Bertness, K. A., Hickernell, R. K., Wang C. M., and Splett, J. D. (2003). Accuracy of AlGaAs growth rates and composition determination using RHEED oscillations. Journal of Crystal Growth. 251(1-4): 73-79. DOI: https://doi.org/10.1016/S0022-0248(03)00840-6
Henini, M. (1993). Semiconductor lasers: An overview part I. III-Vs Review. 6(5): 50-53. DOI: https://doi.org/10.1016/0961-1290(93)90111-Z
Hu, X., Li, G., and Yu, J. C. (2010). Design, fabrication, and modification of nanostructured semiconductor materials for environmental and energy applications. Langmuir. 26(5): 3031-3039. DOI: https://doi.org/10.1021/la902142b
Kyriienko, O., Kavokin, A. V., and Shelykh, I. A. (2013). Superradiant terahertz emission by dipolaritons. Physical Review Letters. 111(17): 176401. DOI: https://doi.org/10.1103/PhysRevLett.111.176401
Lastras-Martínez, L. F., Lastras-Martínez, A., and Balderas-Navarro, R. E. (1993). A spectrometer for the measurement of reflectance-difference spectra. Review of Scientific Instruments. 64(8): 2147-2152. DOI: https://doi.org/10.1063/1.1143952
Miller, R. C. and Kleinman, D. A. (1985). Excitons in GaAs quantum wells. Journal of Luminescence. 30(1-4): 520-540. DOI: https://doi.org/10.1016/0022-2313(85)90075-4
Mishurnyi, V. A. y Lastras-Martínez, A. (2009). Láseres de Semiconductor. México: Editorial Universitaria Potosina. 163 Pp.
Moebs, W., Ling, S. J. y Sanny, J. (2021). Física Universitaria. 9.5 Teoría de bandas de los sólidos, en openstax. [En línea]. Disponible en: https://openstax.org/books/f%C3%ADsica-universitaria-volumen-3/pages/9-5-teoria-de-bandas-de-los-solidos. Fecha de consulta: 1 de enero de 2023.
Morkoc, H. (1982). Influence of MBE Growth conditions on the properties of AlxGa1-xAs/GaAs heterostructures. Journal de Physique Colloques. 43(C5): 209-220. DOI: https://doi.org/10.1051/jphyscol:1982525
Ohring, M. (2002). Materials science of thin films, deposition and structure. California: Academic Press. 794 Pp. DOI: https://doi.org/10.1016/B978-012524975-1/50012-4
Ozturk, O., Ozturk, E., and Elagoz, S. (2018). The effect of barrier width on the electronic properties of double GaAlAs/GaAs and GaInAs/GaAs quantum wells. Journal of Molecular Structure. 40(2): 471-476. DOI: https://doi.org/10.17776/csj.520766
Rosenberg, I., Liran, D., Mazuz-Harpaz, Y., West, K., Pfeiffer, L., and Rapaport, R. (2018). Strongly interacting dipolar-polaritons. Science Advances. 4(10): eaat8880. DOI: https://doi.org/10.1126/sciadv.aat8880
Ruiz-Cigarrillo, O., Lastras-Martínez, L. F., Cerda-Méndez, E. A., Flores-Rangel, G., Bravo-Velazquez, C. A., Balderas-Navarro, R. E., ..., and Santos, P. V. (2021). Optical anisotropies of asymmetric double GaAs (001) quantum wells. Physical Review B. 103(3): 035309. DOI: https://doi.org/10.1103/PhysRevB.103.035309
Sands, D. E. (1993). Introducción a la cristalografía. España: Editorial Reverté. 176 Pp.
Seedhouse, A., Wilkes, J., Kulakovskii, V. D., and Muljarov, E. A. (2019). Terahertz radiation of microcavity dipolaritons. Optics Letters. 44(17): 4339-4342. DOI: https://doi.org/10.1364/OL.44.004339
Serafin, P., Byrnes, T., and Kolmakov, G. V. (2020). Driven dipolariton transistors in Y-shaped channels. Physics Letters A. 384(34): 126855. DOI: https://doi.org/10.1016/j.physleta.2020.126855
Sivalertporn, K. (2016). Effect of barrier width on the exciton states in coupled quantum wells in an applied electric field. Physics Letters A. 380(22-23): 1990-1994. DOI: https://doi.org/10.1016/j.physleta.2016.04.002
Sivalertporn, K., Mouchliadis, L., Ivanov, A. L., Philp, R., and Muljarov, E. A. (2012). Direct and indirect excitons in semiconductor coupled quantum wells in an applied electric field. Physical Review B. 85(4): 045207. DOI: https://doi.org/10.1103/PhysRevB.85.045207
Tapia, L. E. y Santiago-García J. G. (2022). ¿Cómo se fabrica un LED? Universitarios Potosinos. 268: 13-19.
Tsao, J. Y. (2002). Light Emitting Diodes (LEDs) for General Illumination, OIDA Optoelectronics Industry Development Association. [En línea]. Disponible en: https://www1.eere.energy.gov/buildings/publications/pdfs/ssl/report_led_november_2002a_1.pdf. Fecha de consulta: 8 de enero de 2023.
Weightman, P., Martin, D. S., Cole, R. J., and Farrell, T. (2005). Reflection anisotropy spectroscopy. Reports on Progress in Physics. 68(6): 1251. DOI: https://doi.org/10.1088/0034-4885/68/6/R01
Weisbuch, C., Nishioka, M., Ishikawa, A., and Arakawa, Y. (1992). Observation of the coupled exciton-photon mode splitting in a semiconductor quantum microcavity. Physical Review Letters. 69(23): 3314-3317. DOI: https://doi.org/10.1103/PhysRevLett.69.3314
Wilkes, J. and Muljarov, E. A. (2017). Excitons and polaritons in planar heterostructures in external electric and magnetic fields: A multi-sub-level approach, Superlattices and Microstructures. 108: 32-41. DOI: https://doi.org/10.1016/j.spmi.2017.01.027
Witham, O., Hunt, R. J., and Drummond, N. D. (2018). Stability of trions in coupled quantum wells modeled by two-dimensional bilayers. Physical Review B. 97(7): 075424. DOI: https://doi.org/10.1103/PhysRevB.97.075424
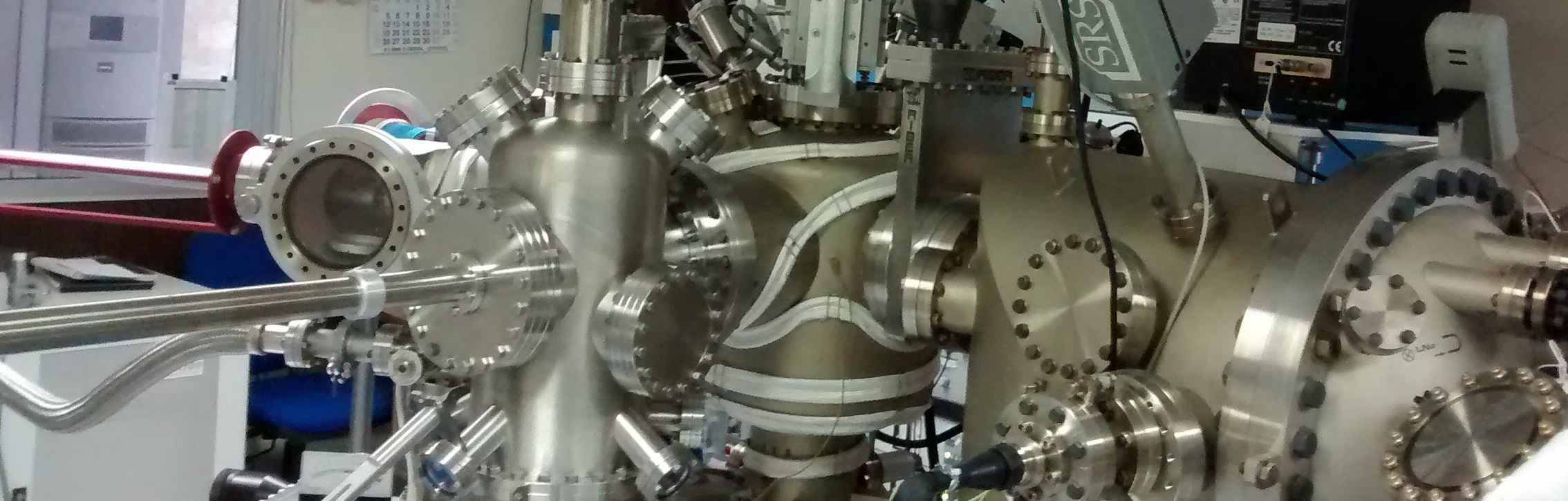
Publicado
Cómo citar
Número
Sección
Categorías
Licencia
Derechos de autor 2022 Universidad Autónoma de Tamaulipas

Esta obra está bajo una licencia internacional Creative Commons Atribución-NoComercial-CompartirIgual 4.0.
Aceptado 2023-01-16
Publicado 2023-01-31